An Integrated Modelling (IM) approach is required to combine the multi-scale and multi-physics aspects for magnetic confinement fusion science. Central to the IM group’s activity is to develop plasma transport modelling integration concepts for the whole fusion device. The regions of interest span across the central plasma-core, with plasma temperatures of the order 100.000.000 °C, and the plasma edge, with complex non-linear physics occurring and strong dissipation facilitating significant pressure drop along the magnetic field towards the divertor region and vessel. It is mandatory to minimize the temperature of the plasma at the point-of-contact with the wall to an acceptable level (i.e., “room-temperature” at several hundreds of °C). The concept of plasma detachment allows to keep the impinging heat-flux densities stable below 10MW/m2 and is a crucial operational constraint in future fusion reactors to avoid melting of the plasma-facing components (e.g. with tungsten as first wall material). The remaining heat-flux densities are still remarkable - rarely seen as for example in atmospheric re-entries of rockets or solar power towers – but manageable by means of material science and technology in a nuclear environment.
The IM group’s activities encompass numerical simulations employing various numerical schemes for relevant plasma- and neutral transport regimes. For example, different levels of sophistication (or flavors) for similar problems exist in the field of plasma edge and divertor physics. A validation process is required to make higher-fidelity codes like SOLPS-ITER (c.f. fig. 1) to adequately interpret and understand in existing tokamak devices e.g. the transition into a controllable high-density, highly-dissipative divertor regime (governed by plasma-neutral interactions, line-emission of impurities and plasma-wall interactions). By means of a validation step-ladder approach, uncertainties in the predictability of high-fidelity codes can be minimized.
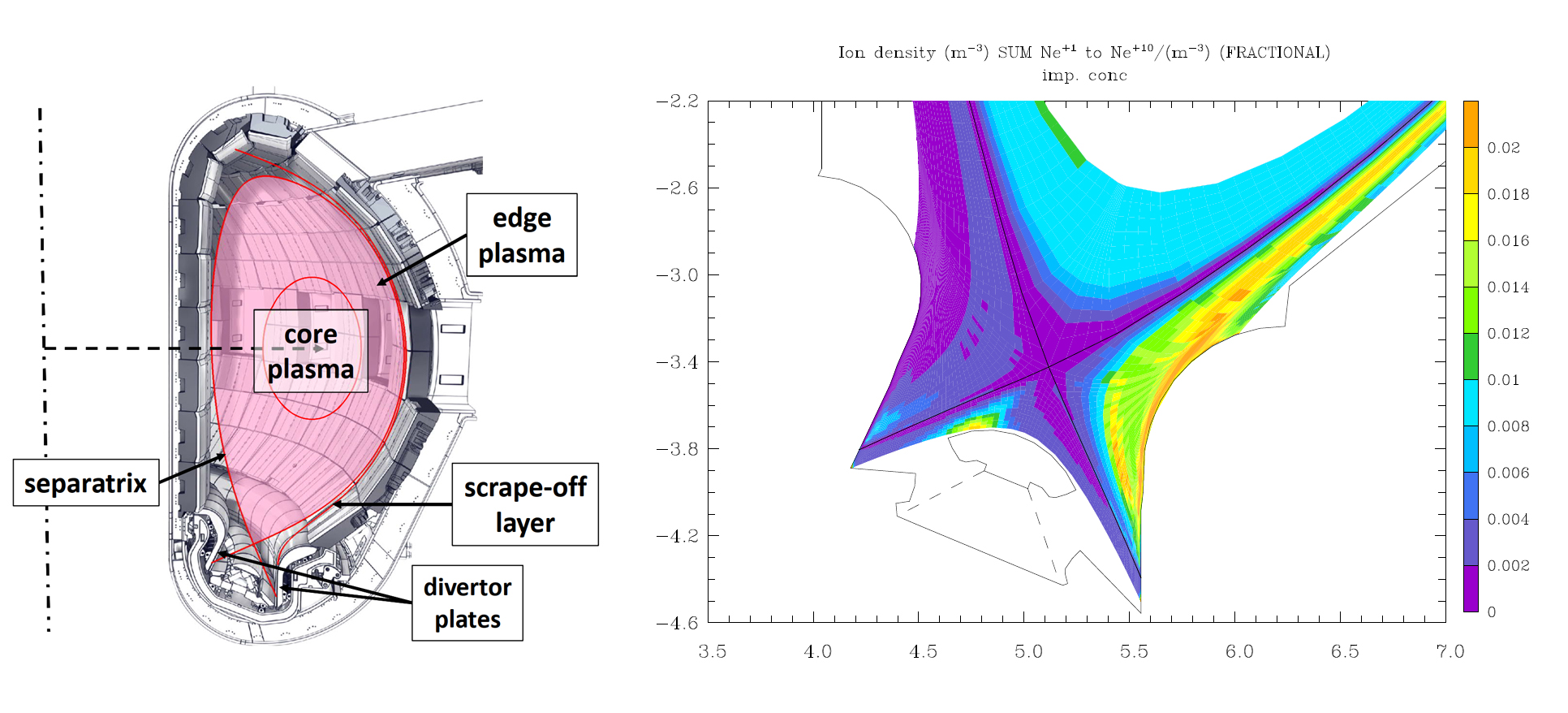
Coupling validated edge-transport models with core-transport models that evolve the performance of the turbulent driven main-plasma transport upstream, must represent the change of transport regimes from closed to open magnetic field lines in the hand-over region (the so-called “separatrix”). This often requires again a reduction in the overall level of fidelity as otherwise a full-device simulation would be then numerically unattractive with respect to numerical convergence times. A multi-fidelity approach is thus required, that provide a) suitable and fast models for plasma-simulators (e.g. as being developed in EUROfusion for ITER and DEMO), and b) still provide important quantities of interest, e.g. the dynamics for plasma control schemes on a reasonable time-scale. New concepts that allow for an adequate reduction of the full-system nowadays can also be facilitated by model surrogates. Recent advances in the employment of Artificial Intelligence (AI) and Machine Learning approaches (ML) seem to be promising (c.f. fig.2) and are central part of the group’s activities, also in collaboration with TU/e and EUROfusion.
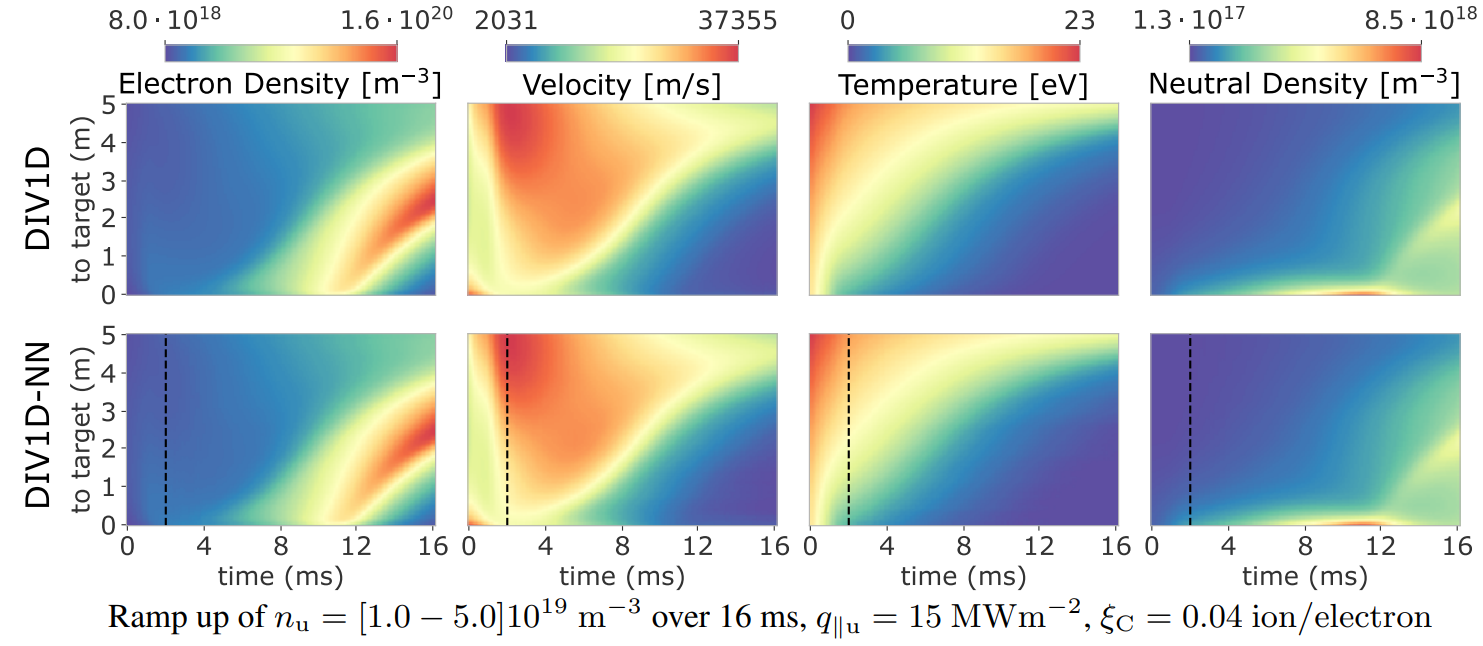