Group leader: Dr. Mihalis Tsampas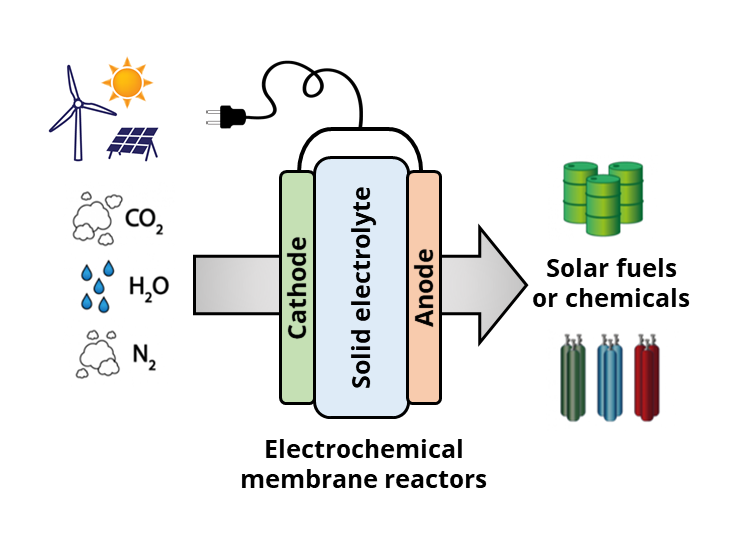
CEPEA research is focused on the area of electrochemical membrane reactors for the production of solar fuels and the electrification of chemical industry. We employ various types of polymeric and ceramic ion exchange conductors that allow us to couple renewable energy-driven processes to a wide range of applications such as water electrolysis, electro-fixation of nitrogen and carbon dioxide, and batteries. In terms of materials synthesis, we follow nanostructuring pathways either for increasing the active surface area of electrocatalysts or for enabling atomic level control of the exposed sites. We are also actively involved in the development of novel approaches, where electrochemical routes are assisted by light or plasma in order to overcome inherent material or process limitations.