So-called plasmonic sensors of noble metal nanoparticles follow how electrical charge is transfered during chemical reactions and energy conversion processes. In a recent paper in the Journal of Physical Chemistry C, DIFFER researchers Matteo Parente and Andrea Baldi together with the Jennifer Dionne group at Stanford University delivered distinct proof that these sensors work in an entirely different way than presumed before. Baldi and Parente: ‘We think this will lead to a whole new level of understanding of photochemical reactions.’
To efficiently convert sunlight into electrical currents or chemical products, it is of vital importance to understand how electric charges produced by the absorption of sunlight in a semiconductor are transferred to metallic contacts and catalysts. The transfer of electrical charges to and from metals can be probed using surface plasmon resonances. These are light-driven oscillations of the free electrons at the surface of a metal nanoparticle. Such resonances are extremely sensitive to minor changes in the particle’s surroundings and can be used for example to detect how hydrogen atoms are absorbed by a metal nanoparticle.
Impossible conclusions
‘Up until now, most researchers interpreted the behavior of these plasmonic sensors by only looking at the spectral shifts of their resonances, without taking too much into account changes in their “sharpness” and intensity,’ Parente and Baldi explain. This often led to the conclusion that tens of thousands of electrons can be exchanged between these metal nanoparticles and their surroundings. ‘In many cases, however, this is impossible on the basis of simple electrostatic considerations. A new interpretation is needed to explain the observed optical effects.’
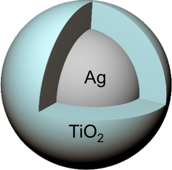
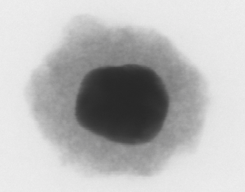
Narrow size distribution
To find out how a redistribution of charges influences the surface plasmon resonances, the DIFFER researchers studied colloidal suspensions of spherical nanoparticles, consisting of a metal core made out of either silver or gold, surrounded by a thin shell of titanium dioxide. ‘The synthetic procedure we used enabled us to produce nanoparticles with narrow size distributions and accurately controlled shell thickness. That way we could come to strong conclusions from optical measurements on the resonance shift,’ explains Baldi.
Blueshift caused by swelling
The suspended particles were illuminated with UV light, which induces the formation of negative electrons and positive holes in the semiconductor shell. The holes are taken up by the surrounding solvent, and the electrons accumulate in the titanium dioxide shell. The measured extinction of the resulting plasmon resonances showed not only the expected shift to shorter wavelengths, and thus higher energies, but also an increase in peak intensities and a narrowing of the resonance peak. The latter two observed effects can only be explained when the electrons are not transported to and accumulated in the metal, as was previously thought. ‘On the contrary, the electrons stay in the titanium dioxide shells, where they remain trapped at structural defects. As a result of this, the shells become highly hydrophilic. This induces an uptake of solvent in its cores, leading to an increase in thickness which subsequently results in the observed blueshift in the spectra,’ states Baldi.
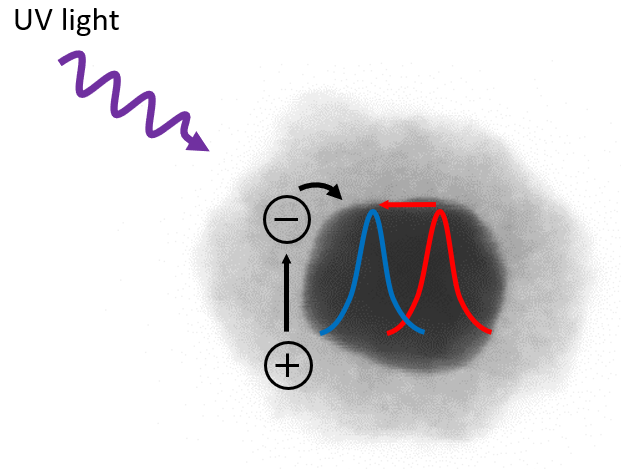
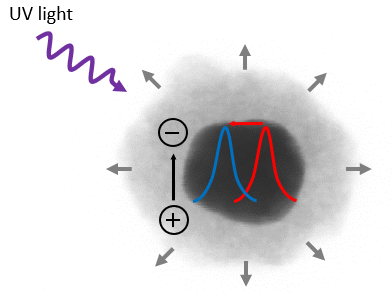
Advanced sensors and plasmon-activated catalysis
‘What I find particularly exciting is that these controlled plasmonic nanoparticles allow us to detect extremely small chemical signals using simple optical measurements,’ states Parente. While the presented work sheds a new light on the mechanism of operation of plasmonic sensors, currently the group at DIFFER is trying to control the “sharpness” of these plasmon resonances. This aspect is in fact closely related to the recent discovery that metal nanoparticles display catalytic properties that can be modified by shining light upon them. One of the explanations for this enhanced catalytic activity is that plasmon resonances can inject electrons into molecules at the surface of the metal nanoparticles. This process, which could explain the observed light-driven catalytic activity of metal nanoparticles, is expected to lead to broader plasmon resonances. Measuring the “sharpness” of these resonances under specific chemical conditions can therefore help understand how plasmon catalysts actually work.
What does this new understanding mean for the plasmonic sensing field? ‘We strongly urge our colleagues to look beyond resonance shifts and take all experimental evidence into account, since that will lead to a whole new level of understanding of photochemical reactions. This in-depth knowledge is crucial for the application of plasmonics to chemical sensing, photocatalysis, and solar energy conversion.’
Equilibration of Photogenerated Charge Carriers in Plasmonic Core@Shell Nanoparticles, Matteo Parente, Sassan Sheikholeslami, Gururaj V. Naik, Jennifer A. Dionne, Andrea Baldi, Journal of Physical Chemistry C, DOI: 10.1021/acs.jpcc.8b05003
Go to the News page.